Timisoara_Med 2020, 2020(1), 8; doi:10.35995/tmj20200108
Review
Angiotensin-Converting-Enzyme 2 and SARS-CoV2: A Dangerous Liaison
1
Department of Functional Sciences—Pathophysiology, Faculty of Medicine, “Victor Babeș” University of Medicine and Pharmacy, Eftimie Murgu Sq. no. 2, 300041 Timișoara, Romania; sturza.adrian@umft.ro
2
Center for Translational Research and Systems Medicine, Faculty of Medicine, “Victor Babeș” University of Medicine and Pharmacy, Eftimie Murgu Sq. no. 2, 300041 Timișoara, Romania
3
Department of Biochemistry, Faculty of Medicine, “Victor Babeș” University of Medicine and Pharmacy, Eftimie Murgu Sq. no. 2, 300041 Timișoara, Romania; cmarian@umft.ro
4
Department of Surgery I, Faculty of Medicine, “Victor Babeș” University of Medicine and Pharmacy, Eftimie Murgu Sq. no. 2, 300041 Timișoara, Romania; octavian.cretu@umft.ro
*
Correspondence: daninamuntean@umft.ro
How to cite: Sturza, A.; Marian, C.V.; Muntean, D.M.; Cretu, O.M. Angiotensin-Converting-Enzyme 2 and SARS-CoV2: A Dangerous Liaison. Timisoara Med. 2020, 2020(1), 8; doi:10.35995/tmj20200108.
Received: 21 September 2020 / Accepted: 20 October 2020 / Published: 26 October 2020
Abstract
:The renin–angiotensin–aldosterone system (RAAS) has been recognized as a key player in the complex scenario of cardiovascular regulation. Aside from its role in the cardiovascular diseases, RAAS dysregulation has emerged as a central pathomechanism in the severe acute respiratory syndrome coronavirus 1 (SARS-CoV1) epidemic, dating back to 2002–2004, and the current COVID-19 pandemic with SARS-CoV2, with the latter involving the interaction with angiotensin-converting enzyme 2 (ACE2). ACE2 is the enzyme responsible for Ang 1-7 production that partly counteracts the RAAS effects and promotes nitric oxide synthase activation; moreover, it has also been reported to act as a receptor for both SARS viruses. In the setting of the ongoing COVID-19 pandemic, the SARS–ACE2 interaction is highly debated with respect to both viral infectivity and usage/discontinuation of RAAS medication—ACE inhibitors (ACEi) and angiotensin-receptor blockers (ARBs)—in diagnosed or suspected SARS-CoV2 patients. Since ACE inhibitors and ARBs are largely prescribed in cardiovascular pathology, a better understanding of the interaction between SARS-CoV2 and RAAS is urgently needed. In this review, we will briefly discuss the SARS-CoV2 and ACE2 interaction and why the discontinuation of RAAS medication is unsafe for either diagnosed or suspected SARS-CoV2 patients.
Keywords:
angiotensin-converting-enzyme 2; SARS-Cov2; COVID-19 pandemicIntroduction
The world continues to face the challenge of the COVID-19 pandemic, caused by the SARS-CoV2 virus, which is responsible for acute lung injury and its major complication, acute respiratory distress syndrome (ARDS), respectively [1]. This pathogen interferes with the renin–angiotensin–aldosterone system (RAAS) activity and, more precisely, has a high affinity for angiotensin-converting enzyme 2 (ACE2), an enzyme that is mainly responsible for the conversion of angiotensin II (ATII) into angiotensin 1-7 [2]. During RAAS activation, AT II binds to angiotensin II type 1 receptors (AT1R) with several deleterious effects in the long run, such as increased blood pressure, vasoconstriction, fluid retention, chronic inflammation, and cardiovascular remodeling [3]. The ACE2 protein can be found in two forms, membrane ACE2 (mACE2) and soluble (sACE2). mACE2 is expressed on the surface of lung alveolar epithelial cells, enterocytes, cardiomyocytes, and endothelial cells from virtually all organs [4,5,6] that elicit opposite, anti-inflammatory, vasodilatory effects and a decrease in blood pressure via Ang 1-7 generation [7,8]. An interaction between SARS-Cov2 and mACE2 followed by virus replication will “block” the action of the enzyme through the shedding of ACE2 by means of the A Disintegrin and Metalloprotease (ADAM)17 protease (ADAM—A Disintegrin and Metalloprotease), thus reducing the expression of the former [9]; the same effect was initially reported for SARS-CoV1 [10]. Important, sACE2 will remain active, not being influenced by the shedding process. As a consequence of lower ACE2 activity/expression, excessive ATII levels will promote lung injury, myocarditis, thrombotic events, and the damage of kidneys and the digestive system. It was reported in a small group of 12 patients with pneumonia and infected with SARS-CoV2 that plasma ATII level was significantly increased and correlated with both lung injuries and viral load [11].
In early studies about fatality rates in SARS-CoV2 infection, a high prevalence of comorbidities such as hypertension, cardiovascular disease, diabetes, malignancies and chronic pulmonary disease was reported [12,13,14]. Notably, patients with chronic obstructive pulmonary disease had a fourfold higher risk of developing COVID-19 [15]. Moreover, elderly patients had an increased susceptibility for this infection, as has been recently shown [14].
Since most patients with cardiovascular and metabolic diseases are treated with ARBs or ACEi, a major concern was raised about the safety and/or persistent beneficial effects of these drugs in SARS-CoV2-infected patients. It was suggested that the interaction between SARS-CoV2 and ACE2 is an important factor for disease aggravation [16,17]. Currently, there are several ongoing clinical trials that investigate the effects of ACEi and ARBs usage in the setting of SARS-CoV2 infection (Table 1). A couple of these studies already concluded that ARBs and ACEi do not aggravate morbidity and mortality in COVID-19 patients with cardiovascular diseases [18] and strongly recommended treatment continuation if indicated [19].
A better understanding of the interaction between SARS-CoV2 and RAAS components is needed for the appropriate therapeutic management of these patients.
ACE2 and RAAS Interaction
ACE2 is a transmembrane glycoprotein (mono-carboxypeptidase), a homolog of ACE (40% identic) [8,14], which converts ATII into its protective metabolite, Ang 1-7. Furthermore, ACE2 can convert angiotensin I into Ang 1-9, which will be further transformed by ACE and ACE2 into Ang 1-7 [8,20]. By all these effects, ACE2 can suppress the activity of RAAS and reduce vasoconstriction and cardiovascular remodeling [21].
Normally, AT1R and ACE2 interact and form complexes on the cellular surface [22]. High levels of ATII will separate and release ACE2 (shedding), a process activated by the metalloproteinase ADAM17 (upregulated by ATII) [9,22]. Even though the process of shedding represents an important mechanism of ACE2 regulation, the amount of the soluble form of ACE2 is small [23]. Interestingly, it was reported that SARS-CoV2 can be blocked by the recombinant soluble ACE2 [24], which might represent a therapeutic option for SARS-CoV2 as well; in this respect, a pilot trial (NCT04335136) has been launched (Table 1). ACE2 represents an important regulatory enzyme of RAAS not only by the reduction in ATII levels, but also because its product—Ang 1-7—has specific effects by acting on the recently discovered receptor Mas (Mas oncogene product) [6,7]. The interaction with this receptor will block AT1R and activate nitric oxide synthase (NOS) with NO production and the suppression of ATII effects [25]. Importantly, Ang 1-7 is able to have a crosstalk interaction with the angiotensin type 2 receptor (AT2R) and bradykinin type 2 receptor (BK2R) [3,26]. Alterations in the Ang 1-7 levels are associated with hypertension, myocardial hypertrophy, myocardial infarction, liver disease and chronic kidney disease [27].
The crucial role of ACE2 in cardiac regulation was firstly described in experimental models. Accordingly, ACE2 deficiency in rodents leads to increased levels of ATII, oxidative stress, inflammation, and hypertension through AT1R activation. ACE2 knockout mice developed increased local cardiac ATII, high oxidative stress, neutrophilic infiltration and pathological hypertrophy [28]. An elevated activity and ACE2 gene expression in pericytes (as compensatory mechanisms) were reported in patients with heart failure and were correlated with severity of the disease [29].
In addition, ARB and ACEi were able to increase ACE2 activity and mRNA gene expression in the rat heart. To date, no data about such an increase at the tissue level were reported in humans; however, ongoing studies are investigating the effects of RAAS medication on circulating ACE2 levels.
The dual effect of RAAS activation is depicted in Figure 1.
SARS-CoV2–ACE2 Interaction
SARS-CoV2 is a pathogen which shares many similarities with SARS-CoV1, including 76% of spike (S) protein sequence identity [30] and an increased affinity for ACE2 (10–20-fold higher than that of SARS-CoV1) [31].
In the heart and lungs, binding of SARS-CoV2 to ACE2 (which acts as a functional receptor) will lead to ACE2 internalization [4,6]. After the binding and endocytosis of SARS-CoV2, ACE2 expression will decrease, with a subsequent increase in ATII accumulation. Importantly, ACE2 expression can be directly reduced by viral replication [32] or through the activation of ADAM17, the enzyme responsible for ACE2 shedding and inactivation (Figure 2).
The “liaison” between SARS-CoV2 and ACE2 receptors is mediated by the spike (S) viral glycoprotein from the viral envelope that is also responsible for the “corona”-like appearance of the virus (Figure 2). The binding process is facilitated by transmembrane protease serine 2 (TMPRSS2), a membrane protease important for the connection between SARS-CoV2 and ACE2 with subsequent viral entry inside the cells (Figure 2) [33].
Genetic susceptibility can be responsible for the rapid spreading of SARS-CoV2. More specifically, it was observed that ACE2 polymorphism (p.Arg514Gly in the African/African-American population) is associated with pulmonary and cardiovascular states, and TMPRSS2 polymorphism (p.Val160Met—rs12329760) can be responsible for the genetic susceptibility to SARS-CoV2 infection (including risk factors such as cancer and male patients) [34].
ACE2 represents the “entry door” for both SARS-CoV1 and SARS-COv2. Indeed, in an experimental model of SARS-CoV1 infection in mice, viral entry facilitated the increased expression of ACE2 [35]. Conversely, in ACE2 knockout mice, the burden of infection with SARS-CoV1 was significantly reduced [4].
On the other hand, ACE2 exerts beneficial effects via the degradation of ATII into Ang 1-7. Suppression of activity/expression of ACE2 will lead to high ATII levels with lung and cardiovascular consequences, an observation reported in a group of patients with SARS-CoV2 infection where plasma levels of ATII were correlated with both the viral load and these types of injuries [11]. Notably, correlations of ATII levels with the viral load and disease severity were also reported in other conditions such as influenza H5N7 and respiratory syncytial viral infections [36,37].
SARS-CoV2 Infection, ACE2 and ACEi/ARB Treatment
Several studies reported that RAAS medication (ACEi and ARB) can increase the membrane ACE2 expression in the cardiovascular system [38,39]. Since ACE2 is the receptor that facilitates SARS-CoV2 entry, treatment with these drugs in patients with hypertension and heart failure was suspected to elicit harmful effects in the setting of SARS-CoV2 infection [17]. However, this hypothesis was not confirmed, since the beneficial effects of ARB via the blockade of ATII action on AT1R appear to prevail [40].
The current hypotheses regarding the dual effect of RAAS inhibition in the presence of SARS-CoV2 infection are depicted in Figure 3.
It has been previously reported in the literature that the SARS-CoV2 infection is more severe in patients with cardiovascular diseases (hypertension, myocardial injury, cardiomyopathy) [41,42], conditions where RAAS medication provides indubitable protection. Thus, discontinuation of these drugs could increase the propensity for decompensation in high-risk patients. When considering the opposite effects on ACE2, i.e., upregulation of ACE2 (induced by ARB and ACEi) vs. downregulation of ACE2 (induced by SARS-CoV2), it is most probably more advantageous to have higher rather than lower enzymatic levels; thus, more ACE2 will be available to limit the ATII effects. In animal studies, ARBs and ACEi showed clearly beneficial effects [4] and a decrease in ACE2 was already reported to be responsible for the maladaptive ventricular remodeling after myocardial infarction [43].
Importantly, no data are currently available in the literature to support the hypothesis that ACEi or ARBs increase the infection rates and/or the severity of the lesions in COVID-19 patients. Therefore, these drugs represent important therapeutic options, if indicated, in the presence of SARS-CoV2 infection. There are animal studies that clearly present the beneficial effects of ARBs on lung injury in SARS-CoV2-infected mice [4].
There are both ongoing and finalized clinical trials (Table 1) that address the effects of RAAS medication in COVID-19 patients, in addition to vaccines and antiviral drugs. Accordingly, the Valsartan for Prevention of Acute Respiratory Distress Syndrome in Hospitalized Patients With SARS-CoV2 (COVID-19) Infection Disease (PRAETORIAN-COVID) trial (NCT04335786, Table 1) investigates the effect of valsartan in SARS-CoV2-infected patients [44]. Other trials investigate the effects of losartan in COVID-19 patients requiring or not requiring hospitalization (NCT04312009, NCT04311177, Table 1). The Angiotensin Receptor Blockers and Angiotensin-Converting Enzyme Inhibitors and Adverse Outcomes in Patients With COVID19 (BRACE-CORONA) trial showed that interruption of ACEi/ARBs for 30 days is not beneficial for COVID-19 patients and the recommendation is to continue the treatment for those with therapeutic indication [19].
Novel Therapeutic Strategies: Recombinant ACE2 and Regulation of Protease Activity
Severe cases of SARS-CoV2 infection are more common in men than in women and in adults/the elderly than in children [45]. Furthermore, the death rate is sex-dependent, being significantly higher in men compared to women [46]. The underlying mechanisms have not been elucidated so far, but, among the contributing factors, smoking, impaired immune response, and the regulation of TMPRSS2 and ADAM17 have been incriminated [9,47]. Moreover, differences in mACE2 expression associated with high AngII levels, ADAM17 activity and increased mACE2 shedding were reported [48], particularly in males [49]. Additionally, it was observed that high levels of sACE2 represent a higher risk factor for severe COVID-19 in adults > children and in men > women [48,50]. In this respect, in two groups of COVID-19 patients with heart failure, sACE2 was found to be higher in men vs. women, an observation that might explain the increased rate of death in men [50].
Regarding SARS-CoV2 treatment, an interesting therapeutic possibility is represented by recombinant ACE2 (Figure 4). The beneficial effects of recombinant ACE2 were demonstrated in animal models of acute lung failure [51]. In this respect, a clinical trial that uses circulating soluble recombinant ACE2 to bind SARS-CoV2 and to prevent its connection with membrane-bound ACE2 is ongoing (NCT04335136, Table 1).
As mentioned before, the process of binding SARS-CoV2 to ACE2 is regulated by two membrane proteases, ADAM17 and TMPRSS2. Their inhibition currently represents the newest therapeutic approach (Figure 4). TMPRSS2 has a crucial role in SARS-CoV2 infection (and also in influenza A virus infection); interestingly, the protease has been reported to be upregulated by androgen hormones, e.g., in prostate cancer [52]. A Japanese trial that investigates the efficiency of two TMPRSS2 inhibitors (nafamostat and camostat) in the treatment of COVID-19 infection is ongoing [53,54].
Conclusions
ACE2, the enzyme responsible for the conversion of ATII into Ang 1-7, also acts as a receptor for SARS-CoV2, the currently ongoing and pandemic-causing coronavirus. ACE2 expression is increased in response to ACEi and ARBs, and there have been concerns regarding their usage in SARS-CoV2 patients. Several clinical trials are ongoing to test the safety and beneficial effects of RAAS medication (ACEi, ARBs) as well as new classes of drugs (recombinant human ACE2 and inhibitors of proteases ADAM17 and TMPRSS2). Until new data are available, the continuation of RAAS medication is recommended in patients suspected or confirmed to have SARS-CoV2 infection.
Author Contributions
Conceptualization, A.S., D.M.M, C.V.M. and O.M.C.; writing—original draft preparation, A.S., D.M.M., C.V.M. and O.M.C.; writing—review and editing, A.S., D.M.M., C.V.M. and O.M.C.; supervision, D.M.M. and O.M.C.
Funding
This research received no external funding.
Conflicts of Interest
The authors declare no conflicts of interest.
References
- Guan, W.-J.; Ni, Z.-Y.; Hu, Y.; Liang, W.-H.; Ou, C.-Q.; He, J.-X.; Liu, L.; Shan, H.; Lei, C.-L.; Hui, D.S.C.; et al. Clinical Characteristics of Coronavirus Disease 2019 in China. N. Engl. J. Med. 2020, 382, 1708–1720. [Google Scholar] [CrossRef] [PubMed]
- Li, W.; Moore, M.J.; Vasilieva, N.; Sui, J.; Wong, S.K.; Berne, M.A.; Somasundaran, M.; Sullivan, J.L.; Luzuriaga, K.; Greenough, T.C.; et al. Angiotensin-converting enzyme 2 is a functional receptor for the SARS coronavirus. Nat. Cell Biol. 2003, 426, 450–454. [Google Scholar] [CrossRef] [PubMed]
- Nehme, A.; Zouein, F.A.; Zayeri, Z.D.; Zibara, K. An Update on the Tissue Renin Angiotensin System and Its Role in Physiology and Pathology. J. Cardiovasc. Dev. Dis. 2019, 6, 14. [Google Scholar] [CrossRef] [PubMed]
- Kuba, K.; Imai, Y.; Rao, S.; Gao, H.; Guo, F.; Guan, B.; Huan, Y.; Yang, P.; Zhang, Y.; Deng, W.; et al. A crucial role of angiotensin converting enzyme 2 (ACE2) in SARS coronavirus–induced lung injury. Nat. Med. 2005, 11, 875–879. [Google Scholar] [CrossRef] [PubMed]
- Oudit, G.Y.; Kassiri, Z.; Jiang, C.; Liu, P.P.; Poutanen, S.M.; Penninger, J.M.; Butany, J. SARS-coronavirus modulation of myocardial ACE2 expression and inflammation in patients with SARS. Eur. J. Clin. Investig. 2009, 39, 618–625. [Google Scholar] [CrossRef]
- Zores, F.; Rebeaud, M.E. COVID and the Renin-Angiotensin System: Are Hypertension or Its Treatments Deleterious? Front. Cardiovasc. Med. 2020, 7, 71. [Google Scholar] [CrossRef]
- Hamming, I.; Cooper, M.E.; Haagmans, B.L.; Hooper, N.M.; Korstanje, R.; Osterhaus, A.D.M.E.; Timens, W.; Turner, A.J.; Navis, G.; Van Goor, H. The emerging role of ACE2 in physiology and disease. J. Pathol. 2007, 212, 1–11. [Google Scholar] [CrossRef]
- Donoghue, M.; Hsieh, F.; Baronas, E.; Godbout, K.; Gosselin, M.; Stagliano, N.; Donovan, M.; Woolf, B.; Robison, K.; Jeyaseelan, R.; et al. A Novel Angiotensin-Converting Enzyme–Related Carboxypeptidase (ACE2) Converts Angiotensin I to Angiotensin 1-9. Circ. Res. 2000, 87, E1–E9. [Google Scholar] [CrossRef]
- Palau, V.; Riera, M.; Soler, M.J. ADAM17 inhibition may exert a protective effect on COVID-19. Nephrol. Dial. Transplant. 2020, 35, 1071–1072. [Google Scholar] [CrossRef] [PubMed]
- Lambert, D.W.; A Yarski, M.; Warner, F.J.; Thornhill, P.; Parkin, E.T.; Smith, A.I.; Hooper, N.M.; Turner, A.J. Tumor Necrosis Factor-α Convertase (ADAM17) Mediates Regulated Ectodomain Shedding of the Severe-acute Respiratory Syndrome-Coronavirus (SARS-CoV) Receptor, Angiotensin-converting Enzyme-2 (ACE2). J. Biol. Chem. 2005, 280, 30113–30119. [Google Scholar] [CrossRef]
- Liu, Y.; Yang, Y.; Zhang, C.; Huang, F.; Wang, F.; Yuan, J.; Wang, Z.; Li, J.; Li, J.; Feng, C.; et al. Clinical and biochemical indexes from 2019-nCoV infected patients linked to viral loads and lung injury. Sci. China Life Sci. 2020, 63, 364–374. [Google Scholar] [CrossRef]
- Sanyaolu, A.; Okorie, C.; Marinkovic, A.; Patidar, R.; Younis, K.; Desai, P.; Hosein, Z.; Padda, I.; Mangat, J.; Altaf, M. Comorbidity and its Impact on Patients with COVID-19. SN Compr. Clin. Med. 2020, 2, 1069–1076. [Google Scholar] [CrossRef]
- Singh, A.K.; Gupta, R.; Ghosh, A.; Misra, A. Diabetes in COVID-19: Prevalence, pathophysiology, prognosis and practical considerations. Diabetes Metab. Syndr. Clin. Res. Rev. 2020, 14, 303–310. [Google Scholar] [CrossRef]
- Liu, K.; Chen, Y.; Lin, R.; Han, K. Clinical features of COVID-19 in elderly patients: A comparison with young and middle-aged patients. J. Infect. 2020, 80, e14–e18. [Google Scholar] [CrossRef]
- Zhao, Q.; Meng, M.; Kumar, R.; Wu, Y.; Huang, J.; Lian, N.; Deng, Y.; Lin, S. The impact of COPD and smoking history on the severity of COVID-19: A systemic review and meta-analysis. J. Med. Virol. 2020, 92, 1915–1921. [Google Scholar] [CrossRef]
- Esler, M.; Esler, D. Can angiotensin receptor-blocking drugs perhaps be harmful in the COVID-19 pandemic? J. Hypertens. 2020, 38, 781–782. [Google Scholar] [CrossRef]
- Watkins, J. Preventing a covid-19 pandemic. BMJ 2020, 368, m810. [Google Scholar] [CrossRef]
- Peng, Y.D.; Meng, K.; Guan, H.Q.; Leng, L.; Zhu, R.R.; Wang, B.Y.; A He, M.; Cheng, L.X.; Huang, K.; Zeng, Q.T. [Clinical characteristics and outcomes of 112 cardiovascular disease patients infected by 2019-nCoV]. Zhonghua Xin Xue Guan Bing Za Zhi 2020, 48, E004. [Google Scholar]
- Lopes, R.D.; Macedo, A.V.S.; Silva, P.G.M.D.B.E.; Moll-Bernardes, R.J.; Feldman, A.; Arruda, G.D.S.; De Souza, A.S.; De Albuquerque, D.C.; Mazza, L.; Santos, M.F.; et al. Continuing versus suspending angiotensin-converting enzyme inhibitors and angiotensin receptor blockers: Impact on adverse outcomes in hospitalized patients with severe acute respiratory syndrome coronavirus 2 (SARS-CoV-2)—The BRACE CORONA Trial. Am. Heart J. 2020, 226, 49–59. [Google Scholar] [CrossRef]
- Ferrario, C.M.; Jessup, J.; Gallagher, P.E.; Averill, D.B.; Brosnihan, K.B.; Tallant, E.A.; Smith, R.D.; Chappell, M.C. Effects of renin-angiotensin system blockade on renal angiotensin-(1-7) forming enzymes and receptors. Kidney Int. 2005, 68, 2189–2196. [Google Scholar] [CrossRef]
- Tikellis, C.; Thomas, M.C. Angiotensin-Converting Enzyme 2 (ACE2) Is a Key Modulator of the Renin Angiotensin System in Health and Disease. Int. J. Pept. 2012, 2012, 256294. [Google Scholar] [CrossRef] [PubMed]
- Deshotels, M.R.; Xia, H.; Sriramula, S.; Lazartigues, E.; Filipeanu, C.M. Angiotensin II Mediates Angiotensin Converting Enzyme Type 2 Internalization and Degradation Through an Angiotensin II Type I Receptor–Dependent Mechanism. Hypertension 2014, 64, 1368–1375. [Google Scholar] [CrossRef] [PubMed]
- Lambert, D.W.; Hooper, N.M.; Turner, A.J. Angiotensin-converting enzyme 2 and new insights into the renin–angiotensin system. Biochem. Pharmacol. 2008, 75, 781–786. [Google Scholar] [CrossRef]
- Batlle, D.; Wysocki, J.; Satchell, K. Soluble angiotensin-converting enzyme 2: A potential approach for coronavirus infection therapy? Clin. Sci. 2020, 134, 543–545. [Google Scholar] [CrossRef]
- Ingelfinger, J.R. Angiotensin-converting enzyme 2: Implications for blood pressure and kidney disease. Curr. Opin. Nephrol. Hypertens. 2009, 18, 79–84. [Google Scholar] [CrossRef]
- Tom, B.; Dendorfer, A.; Danser, A.H.J. Bradykinin, angiotensin-(1-7), and ACE inhibitors: How do they interact? Int. J. Biochem. Cell Biol. 2003, 35, 792–801. [Google Scholar] [CrossRef]
- Ribeiro-Oliveira, A., Jr.; Nogueira, A.I.; Pereira, R.M.; Boas, W.W.; Dos Santos, R.A.; Simõoes E Silva, A.C. The renin–angiotensin system and diabetes: An update. Vasc. Health Risk Manag. 2008, 4, 787–803. [Google Scholar] [CrossRef]
- Oudit, G.Y.; Kassiri, Z.; Patel, M.P.; Chappell, M.; Butany, J.; Backx, P.H.; Tsushima, R.G.; Scholey, J.W.; Khokha, R.; Penninger, J.M. Angiotensin II-mediated oxidative stress and inflammation mediate the age-dependent cardiomyopathy in ACE2 null mice. Cardiovasc. Res. 2007, 75, 29–39. [Google Scholar] [CrossRef]
- Chen, L.; Li, X.; Chen, M.; Feng, Y.; Xiong, C. The ACE2 expression in human heart indicates new potential mechanism of heart injury among patients infected with SARS-CoV-2. Cardiovasc. Res. 2020, 116, 1097–1100. [Google Scholar] [CrossRef]
- Wang, Y.; Liu, M.; Gao, J. Enhanced receptor binding of SARS-CoV-2 through networks of hydrogen-bonding and hydrophobic interactions. Proc. Natl. Acad. Sci. USA 2020, 117, 13967–13974. [Google Scholar] [CrossRef]
- Wrapp, D.; Wang, N.; Corbett, K.S.; Goldsmith, J.A.; Hsieh, C.-L.; Abiona, O.; Graham, B.S.; McLellan, J.S. Cryo-EM structure of the 2019-nCoV spike in the prefusion conformation. Science 2020, 367, 1260–1263. [Google Scholar] [CrossRef]
- Verdecchia, P.; Cavallini, C.; Spanevello, A.; Angeli, F. The pivotal link between ACE2 deficiency and SARS-CoV-2 infection. Eur. J. Intern. Med. 2020, 76, 14–20. [Google Scholar] [CrossRef] [PubMed]
- Hoffmann, M.; Kleine-Weber, H.; Schroeder, S.; Krüger, N.; Herrler, T.; Erichsen, S.; Schiergens, T.S.; Herrler, G.; Wu, N.-H.; Nitsche, A.; et al. SARS-CoV-2 Cell Entry Depends on ACE2 and TMPRSS2 and Is Blocked by a Clinically Proven Protease Inhibitor. Cell 2020, 181, 271–280.e8. [Google Scholar] [CrossRef]
- Hou, Y.; Zhao, J.; Martin, W.; Kallianpur, A.; Chung, M.K.; Jehi, L.; Sharifi, N.; Erzurum, S.; Eng, C.; Cheng, F. New insights into genetic susceptibility of COVID-19: An ACE2 and TMPRSS2 polymorphism analysis. BMC Med. 2020, 18, 216. [Google Scholar] [CrossRef]
- Yang, X.-H.; Deng, W.; Tong, Z.; Liu, Y.-X.; Zhang, L.-F.; Zhu, H.; Gao, H.; Huang, L.; Liu, Y.-L.; Ma, C.-M.; et al. Mice transgenic for human angiotensin-converting enzyme 2 provide a model for SARS coronavirus infection. Comp. Med. 2007, 57, 450–459. [Google Scholar]
- Huang, F.; Guo, J.; Zou, Z.; Liu, J.; Cao, B.; Zhang, S.; Li, H.; Wang, W.; Sheng, M.; Liu, S.; et al. Angiotensin II plasma levels are linked to disease severity and predict fatal outcomes in H7N9-infected patients. Nat. Commun. 2014, 5, 3595. [Google Scholar] [CrossRef]
- Gu, H.; Xie, Z.; Li, T.; Zhang, S.; Lai, C.; Zhu, P.; Wang, K.; Han, L.; Duan, Y.; Zhao, Z.; et al. Angiotensin-converting enzyme 2 inhibits lung injury induced by respiratory syncytial virus. Sci. Rep. 2016, 6, 19840. [Google Scholar] [CrossRef]
- Wang, X.; Ye, Y.; Gong, H.; Wu, J.; Yuan, J.; Wang, S.; Yin, P.; Ding, Z.; Kang, L.; Jiang, Q.; et al. The effects of different angiotensin II type 1 receptor blockers on the regulation of the ACE-AngII-AT1 and ACE2-Ang(1–7)-Mas axes in pressure overload-induced cardiac remodeling in male mice. J. Mol. Cell. Cardiol. 2016, 97, 180–190. [Google Scholar] [CrossRef]
- Ishiyama, Y.; Gallagher, P.E.; Averill, D.B.; Tallant, E.A.; Brosnihan, K.B.; Ferrario, C.M. Upregulation of Angiotensin-Converting Enzyme 2 After Myocardial Infarction by Blockade of Angiotensin II Receptors. Hypertension 2004, 43, 970–976. [Google Scholar] [CrossRef]
- Vaduganathan, M.; Vardeny, O.; Michel, T.; McMurray, J.J.; Pfeffer, M.A.; Solomon, S.D. Renin–Angiotensin–Aldosterone System Inhibitors in Patients with Covid-19. N. Engl. J. Med. 2020, 382, 1653–1659. [Google Scholar] [CrossRef]
- Shi, S.; Qin, M.; Shen, B.; Cai, Y.; Liu, T.; Yang, F.; Gong, W.; Liu, X.; Liang, J.; Zhao, Q.; et al. Association of Cardiac Injury With Mortality in Hospitalized Patients With COVID-19 in Wuhan, China. JAMA Cardiol. 2020, 5, 802. [Google Scholar] [CrossRef]
- Zhou, F.; Yu, T.; Du, R.; Fan, G.; Liu, Y.; Liu, Z.; Xiang, J.; Wang, Y.; Song, B.; Gu, X.; et al. Clinical course and risk factors for mortality of adult inpatients with COVID-19 in Wuhan, China: A retrospective cohort study. Lancet 2020, 395, 1054–1062. [Google Scholar] [CrossRef]
- Kassiri, Z.; Zhong, J.; Guo, D.; Basu, R.; Wang, X.; Liu, P.P.; Scholey, J.W.; Penninger, J.M.; Oudit, G.Y. Loss of Angiotensin-Converting Enzyme 2 Accelerates Maladaptive Left Ventricular Remodeling in Response to Myocardial Infarction. Circ. Heart Fail. 2009, 2, 446–455. [Google Scholar] [CrossRef]
- Gommans, D.H.F.; Nas, J.; Pinto-Sietsma, S.-J.; Koop, Y.; Konst, R.E.; Mensink, F.; Aarts, G.W.; Konijnenberg, L.S.; Cortenbach, K.; Verhaert, D.V.; et al. Rationale and design of the PRAETORIAN-COVID trial: A double-blind, placebo-controlled randomized clinical trial with valsartan for PRevention of Acute rEspiraTORy dIstress syndrome in hospitAlized patieNts with SARS-COV-2 Infection Disease. Am. Heart J. 2020, 226, 60–68. [Google Scholar] [CrossRef] [PubMed]
- Chen, J.; Jiang, Q.; Xia, X.; Liu, K.; Yu, Z.; Tao, W.; Gong, W.; Han, J.J. Individual variation of the SARS-CoV-2 receptor ACE2 gene expression and regulation. Aging Cell 2020, 19. [Google Scholar] [CrossRef] [PubMed]
- Gagliardi, M.C.; Tieri, P.; Ortona, E.; Ruggieri, A. ACE2 expression and sex disparity in COVID-19. Cell Death Discov. 2020, 6, 37. [Google Scholar] [CrossRef]
- Guan, W.-J.; Liang, W.-H.; Zhao, Y.; Liang, H.-R.; Chen, Z.-S.; Li, Y.-M.; Liu, X.-Q.; Chen, R.-C.; Tang, C.-L.; Wang, T.; et al. Comorbidity and its impact on 1590 patients with COVID-19 in China: A nationwide analysis. Eur. Respir. J. 2020, 55, 2000547. [Google Scholar] [CrossRef]
- Swärd, P.; Edsfeldt, A.; Reepalu, A.; Jehpsson, L.; Rosengren, B.E.; Karlsson, M.K. Age and sex differences in soluble ACE2 may give insights for COVID-19. Crit. Care 2020, 24, 221. [Google Scholar] [CrossRef]
- Galbadage, T.; Peterson, B.M.; Awada, J.; Buck, A.S.; Ramirez, D.A.; Wilson, J.; Gunasekera, R.S. Systematic Review and Meta-Analysis of Sex-Specific COVID-19 Clinical Outcomes. Front. Med. 2020, 7, 348. [Google Scholar] [CrossRef]
- Sama, I.E.; Ravera, A.; Santema, B.T.; Van Goor, H.; Ter Maaten, J.M.; Cleland, J.G.F.; Rienstra, M.; Friedrich, A.W.; Samani, N.J.; Ng, L.L.; et al. Circulating plasma concentrations of angiotensin-converting enzyme 2 in men and women with heart failure and effects of renin–angiotensin–aldosterone inhibitors. Eur. Heart J. 2020, 41, 1810–1817. [Google Scholar] [CrossRef]
- Imai, Y.; Kuba, K.; Rao, S.; Huan, Y.; Guo, F.; Guan, B.; Yang, P.; Sarao, R.; Wada, T.; Leong-Poi, H.; et al. Angiotensin-converting enzyme 2 protects from severe acute lung failure. Nat. Cell Biol. 2005, 436, 112–116. [Google Scholar] [CrossRef]
- Wambier, C.G.; Goren, A.; Vaño-Galván, S.; Ramos, P.M.; Ossimetha, A.; Nau, G.; Herrera, S.; McCoy, J. Androgen sensitivity gateway to COVID-19 disease severity. Drug Dev. Res. 2020. [Google Scholar] [CrossRef]
- Hoffmann, M.; Schroeder, S.; Kleine-Weber, H.; Müller, M.A.; Drosten, C.; Pöhlmann, S. Nafamostat Mesylate Blocks Activation of SARS-CoV-2: New Treatment Option for COVID-19. Antimicrob. Agents Chemother. 2020, 64. [Google Scholar] [CrossRef]
- Kai, H.; Kai, M. Interactions of coronaviruses with ACE2, angiotensin II, and RAS inhibitors—Lessons from available evidence and insights into COVID-19. Hypertens. Res. 2020, 43, 648–654. [Google Scholar] [CrossRef]
Figure 1.
Renin–angiotensin–aldosterone system (RAAS) components—the balance between angiotensin-converting enzyme (ACE) and ACE2 effects. Ang I = angiotensin I, Ang II = angiotensin II, Ang 1-7 = angiotensin 1-7 peptide, Ang 1-9 = angiotensin 1-9 peptide, ACE = angiotensin I converting enzyme 1, ACE2 = angiotensin I converting enzyme 2, AT1R = angiotensin II receptor type 1, AT2R = angiotensin II receptor type 2, AT4R = angiotensin II receptor type 4, MasR = Mas oncogene (a G-protein–coupled receptor), BK2R = bradykinin type 2 receptor, NO = nitric oxide, NOS = nitric oxide synthase.
Figure 1.
Renin–angiotensin–aldosterone system (RAAS) components—the balance between angiotensin-converting enzyme (ACE) and ACE2 effects. Ang I = angiotensin I, Ang II = angiotensin II, Ang 1-7 = angiotensin 1-7 peptide, Ang 1-9 = angiotensin 1-9 peptide, ACE = angiotensin I converting enzyme 1, ACE2 = angiotensin I converting enzyme 2, AT1R = angiotensin II receptor type 1, AT2R = angiotensin II receptor type 2, AT4R = angiotensin II receptor type 4, MasR = Mas oncogene (a G-protein–coupled receptor), BK2R = bradykinin type 2 receptor, NO = nitric oxide, NOS = nitric oxide synthase.
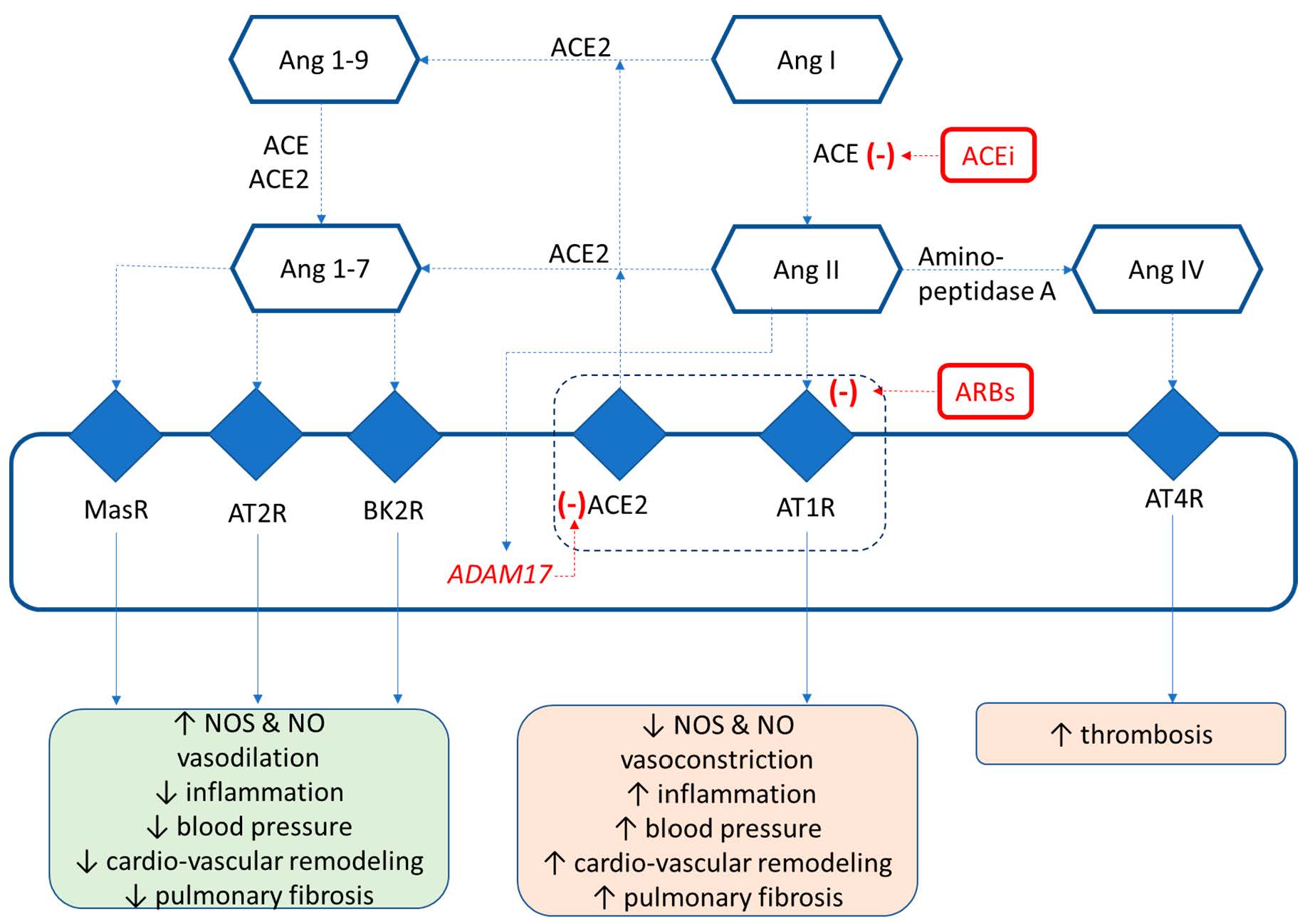
Figure 2.
Mechanisms of SARS-CoV2–ACE2 binding and inactivation.
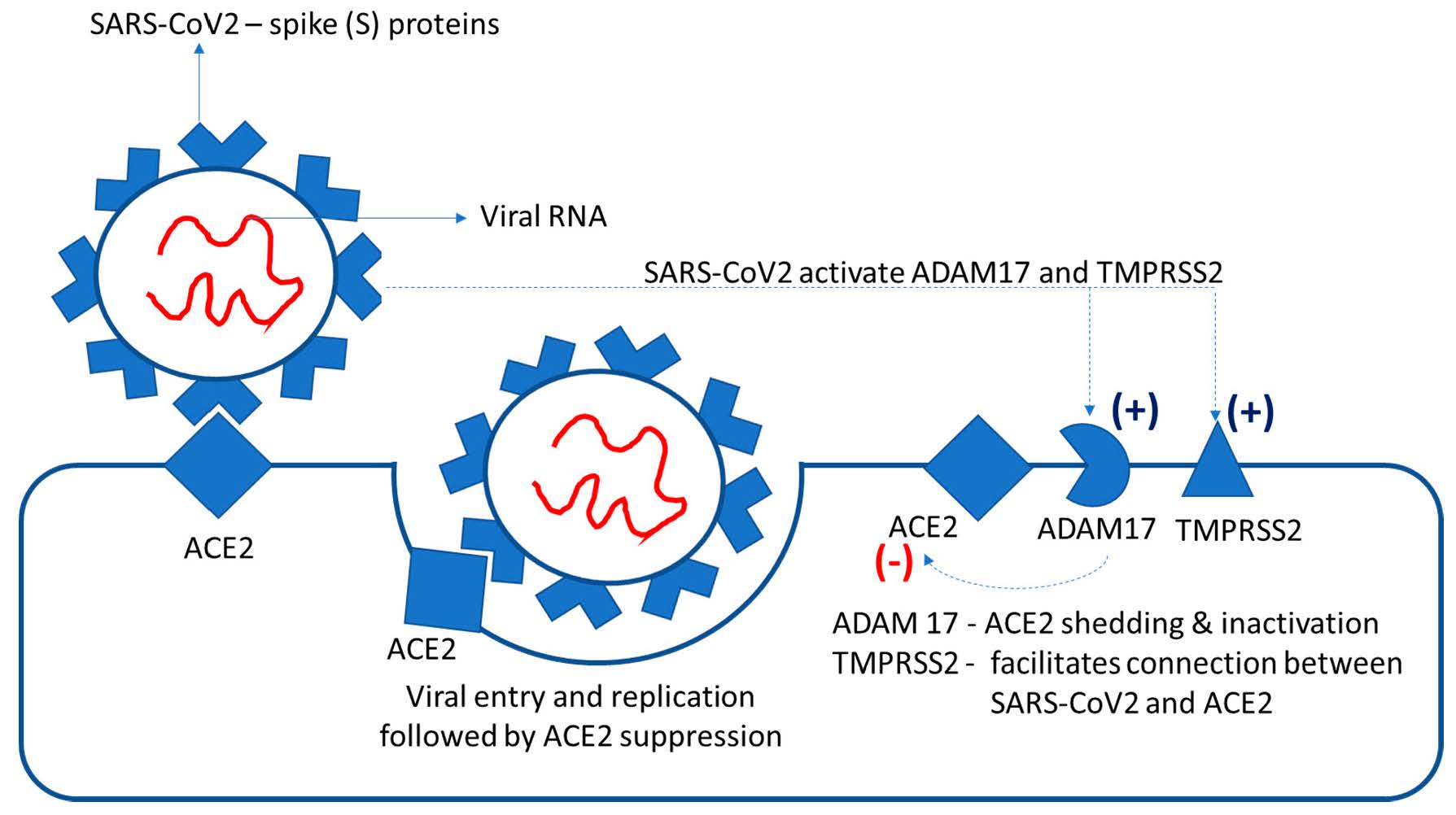
Figure 3.
Current hypotheses regarding the effects of angiotensin-receptor blockers (ARBs) and ACE inhibitors (ACEi) in COVID-19 patients.
Figure 3.
Current hypotheses regarding the effects of angiotensin-receptor blockers (ARBs) and ACE inhibitors (ACEi) in COVID-19 patients.
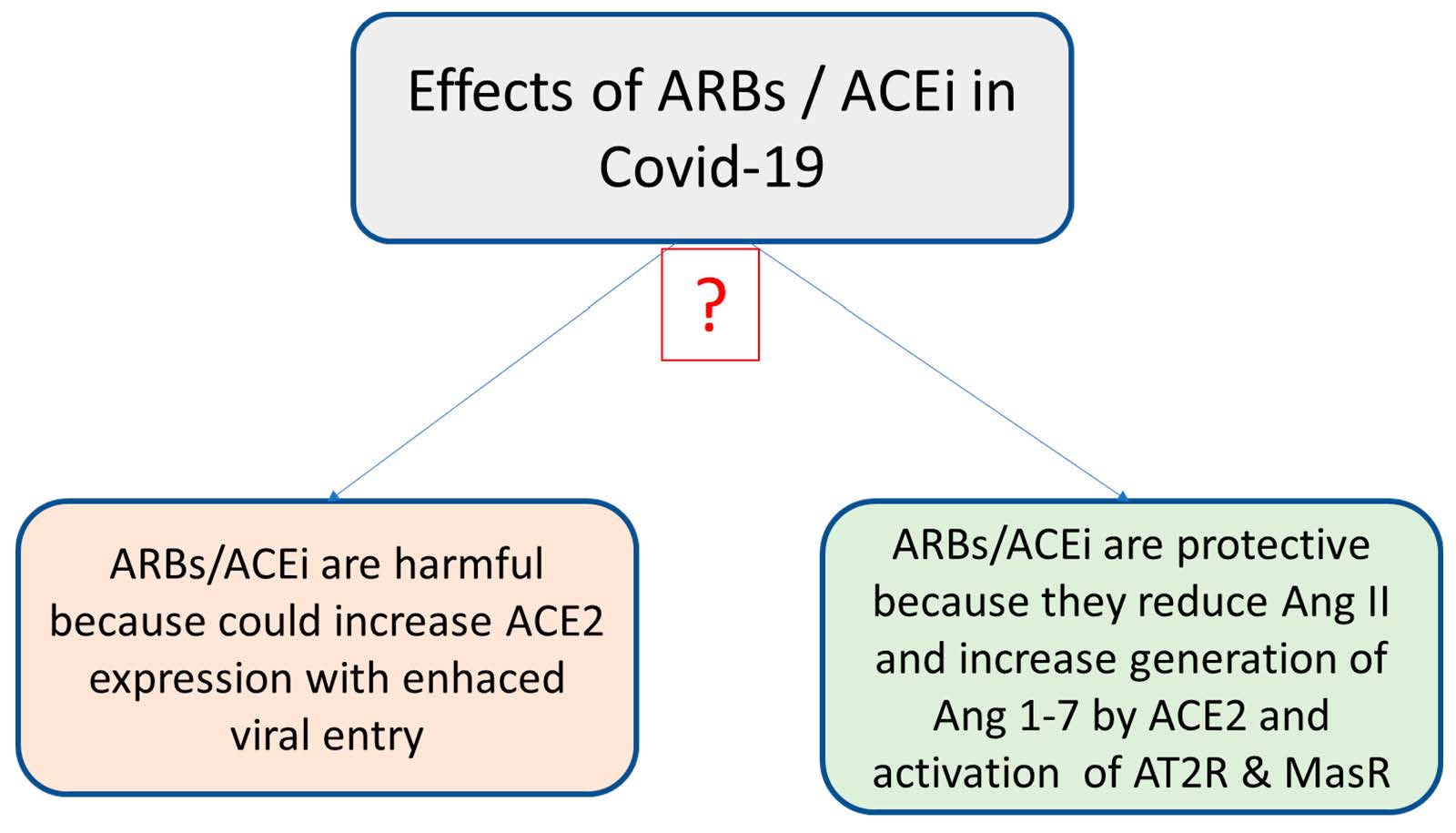
Figure 4.
New therapeutic strategies for preventing SARS-CoV2–ACE2 binding and replication: recombinant ACE2 and inhibitors of proteases A Disintegrin and Metalloprotease (ADAM)17 and transmembrane protease serine 2 (TMPRSS2).
Figure 4.
New therapeutic strategies for preventing SARS-CoV2–ACE2 binding and replication: recombinant ACE2 and inhibitors of proteases A Disintegrin and Metalloprotease (ADAM)17 and transmembrane protease serine 2 (TMPRSS2).
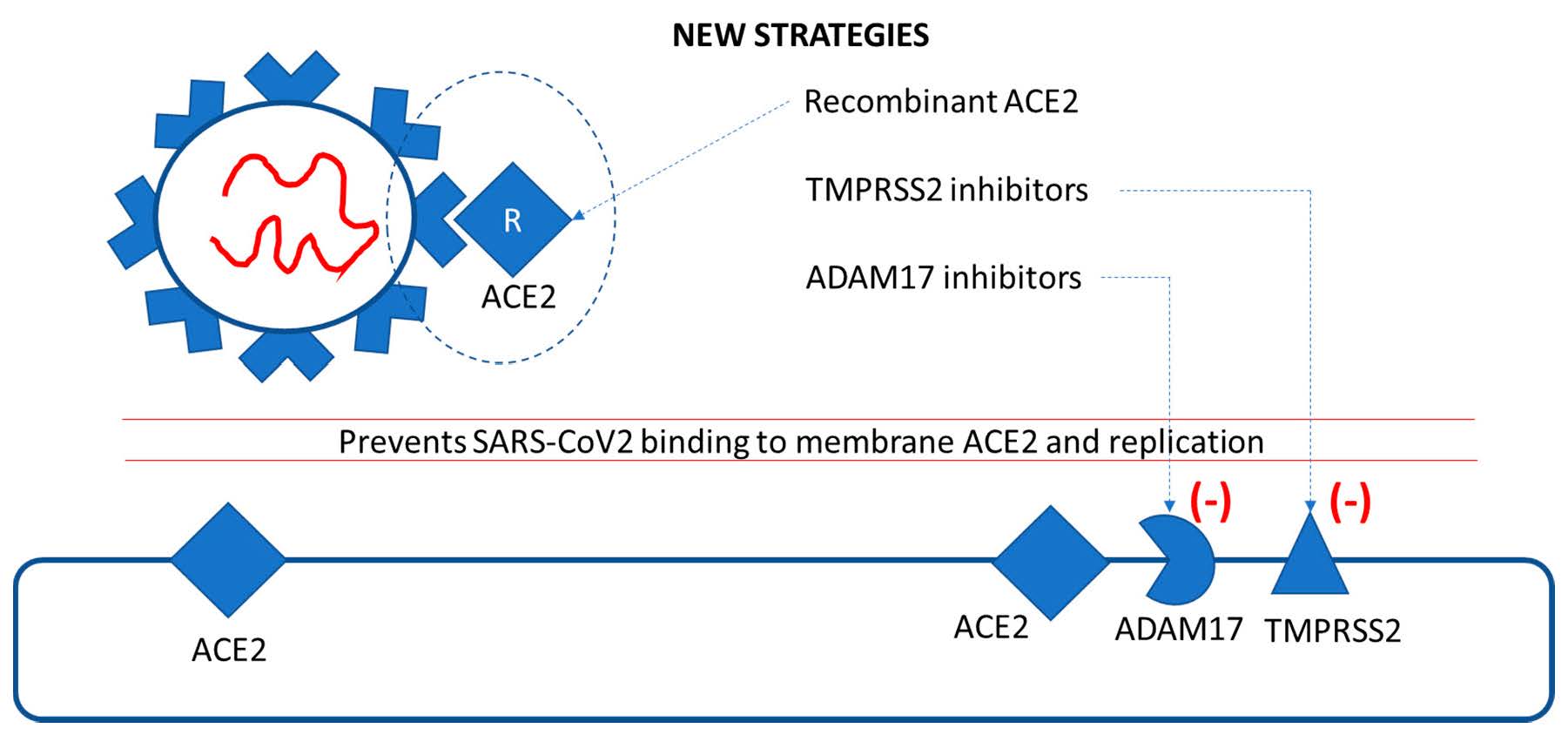
Table 1.
Clinical trials for investigation of treatment with ARBs and ACEi in COVID-19 patients (source: ClinicalTrials.gov).
Table 1.
Clinical trials for investigation of treatment with ARBs and ACEi in COVID-19 patients (source: ClinicalTrials.gov).
Trial Number | Title |
---|---|
NCT04312009 | Losartan for Patients with COVID-19 Requiring Hospitalization |
NCT04311177 | Losartan for Patients with COVID-19 Not Requiring Hospitalization |
NCT04335136 | Recombinant Human Angiotensin-converting Enzyme 2 (rhACE2) as a Treatment for Patients with COVID-19 (APN01-COVID-19) |
NCT04364893 | Angiotensin Receptor Blockers and Angiotensin-Converting Enzyme Inhibitors and Adverse Outcomes in Patients with COVID19 (BRACE-CORONA) |
NCT04338009 | Elimination or Prolongation of ACE Inhibitors and ARB in Coronavirus Disease 2019 (REPLACECOVID) |
NCT04375046 | Recombinant Bacterial ACE2 Receptors—Like Enzyme of B38-CAP Could be Promising COVID-19 Infection—and Lung Injury Preventing Drug Better Than Recombinant Human ACE2 (Bacterial ACE2) |
NCT04318418 | ACE Inhibitors, Angiotensin II Type-I Receptor Blockers and Severity of COVID-19 (CODIV-ACE) |
NCT04353596 | Stopping ACE inhibitors in COVID-19 (ACEI-COVID) |
NCT04357535 | Prognosis of Coronavirus Disease 2019 (COVID-19) Patients Receiving Antihypertensives |
NCT04364893 | Angiotensin Receptor Blockers and Angiotensin-Converting Enzyme Inhibitors and Adverse Outcomes in Patients with COVID19 (BRACE-CORONA) |
NCT04337190 | Impact of Angiotensin II Receptor Blockers Treatment in Patients with COVID 19 (COVID-ARA2) |
NCT04367883 | Influenza Vaccination, ACEI and ARB in the Evolution of SARS-COVID19 Infection |
NCT04329195 | ACE Inhibitors or ARBs Discontinuation in Context of SARS-CoV2 Pandemic |
NCT04519398 | Investigating the Involvement of ACE and Angiotensinogen Genes’ Polymorphism Along with Other Thrombophilic Genotypes in Severe Forms of COVID-19 with/without Thrombotic Events |
NCT04338009 | Elimination or Prolongation of ACE Inhibitors and ARB in Coronavirus Disease 2019 |
NCT04351581 | Effects of Discontinuing Renin–Angiotensin System Inhibitors in Patients with COVID-19 |
NCT04331574 | Renin–Angiotensin System Inhibitors and COVID-19 |
NCT04394117 | Controlled Evaluation of Angiotensin Receptor Blockers for COVID-19 Respiratory Disease (CLARITY) |
NCT04355936 | Telmisartan for Treatment of COVID-19 Patients |
NCT04335786 | Valsartan for Prevention of Acute Respiratory Distress Syndrome in Hospitalized Patients with SARS-CoV2 (COVID-19) Infection Disease (PRAETORIAN-COVID) |
© 2020 Copyright by the authors. Licensed as an open access article using a CC BY 4.0 license.